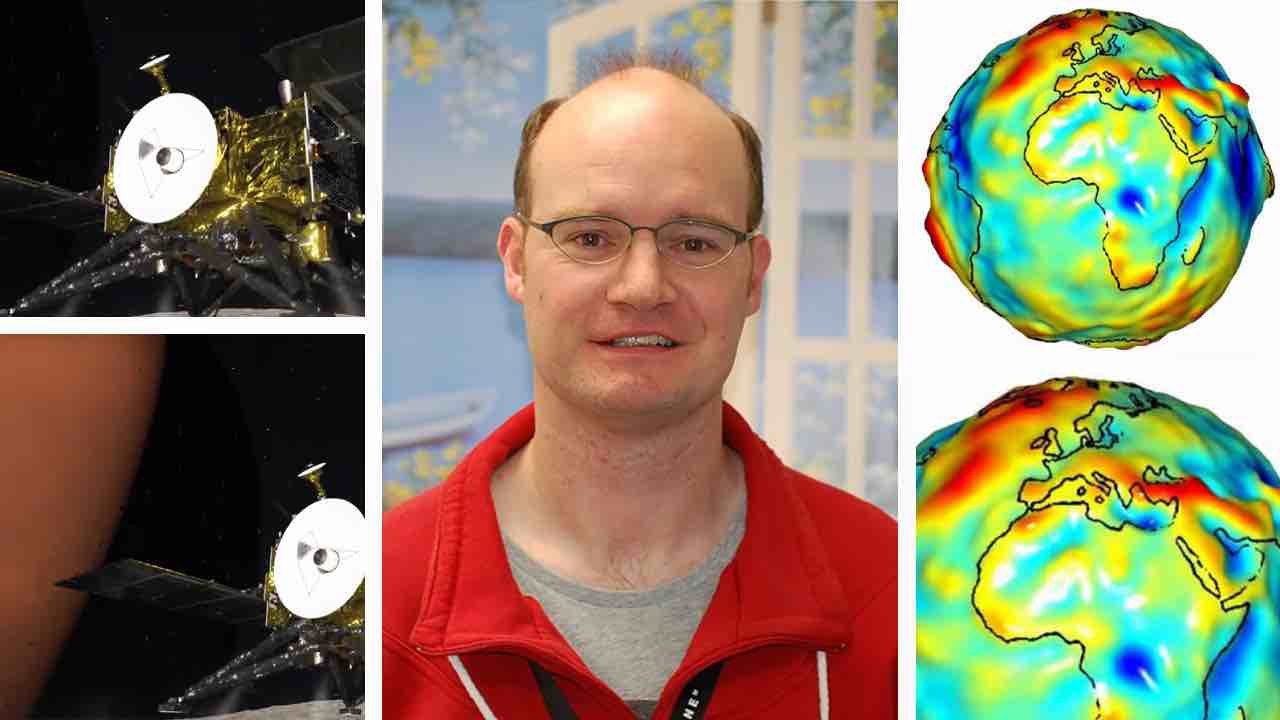
We met Sander J. Goossens, Planetary Scientist at NASA’s Goddard Space Flight Center in Greenbelt (Maryland, USA). He studied the gravity field of various moons and planets of Solar System. Then, he’s participating at NASA’s Juno Mission, with the aim of studying the gravity field of the Galilean moons.
Recently, Goossens was selected by NASA to conduct research using the MMX flight instruments.The JAXA’s Martian Moons Exploration (MMX) mission will be launched in 2024 with the goal of visiting the two moons of Mars Phobos and Deimos. In addition, a lander will land on Phobos and collect samples, which will be brought to Earth in 2029.
In your career you have studied the gravitational field of the Moon and many planets. Why is it important to study the gravitational field? What discoveries have you made?
The gravity field of a celestial body can tell us something about its interior. The best way to do this is seismology, but we only have limited data, apart from the Earth. There is seismology data for the Moon from the Apollo era, and now for Mars from NASA’s InSight mission. The combination of gravity and topography (the shape of a body), together with for example knowledge of a body’s rotation, is also a powerful one, because it can be used to extract information about the interior, such as the structure of the crust and whether the body has a core. We want to know about the interior structure of a body in order to get a better understanding of the formation and evolution of different planets in the solar system.
I have been lucky enough to have worked on several missions that collected new and important data sets. For the Moon, I was involved in the mission that collected the first global data for gravity field determination, which was JAXA’s (Japan Aerospace Exploration Agency) Kaguya mission. Determining the global gravity field of the Moon is difficult because it is always facing the Earth with the same side. The gravity field of a body can be determined by using radio tracking from Earth to a spacecraft in orbit around that body; by determining the spacecraft orbit precisely, local variations in the gravity field can be inferred. For the Moon, we lose that tracking link when the spacecraft orbits the farside. Kaguya achieved global coverage by using two satellites, one serving as a relay to establish a link when the main satellite was over the farside. NASA’s Gravity Recovery and Interior Laboratory (GRAIL) mission then improved our knowledge of the Moon’s gravity field many times over by employing a very precise radio link between two low orbiting spacecraft. When combined with topography data from NASA’s Lunar Reconnaissance Orbiter (LRO), it was found that the crust of the Moon was highly fractured and homogenized due to impacts, among many other science discoveries.
At NASA, I have been involved in many other missions. The Mercury Surface, Space Environment, Geochemistry, and Ranging (MESSENGER) mission was the first to orbit Mercury. Using MESSENGER data we provided improved estimates of the size of its core, and even found evidence that Mercury may have a solid inner core. And using tracking data from Mars spacecraft, we were able to derive an estimate for the density of its crust, indicating that it could be lower than previously assumed.
By studying the gravitational field can we learn more about the space-time fabric? For example, can we know how it moves, whether it is rigid or elastic?
The gravity field of the planets as studied with spacecraft tracking data to determine the interior structure of the body is at different scales than studying the fundamental force of gravity. Nonetheless, because the radio tracking between a spacecraft and tracking station on Earth (such as NASA’s Deep Space Network) is very precise, such data have been used to test theories of gravity. I was involved as a co-author in one such study using MESSENGER data. The study was able to confirm with improved precision the strong equivalence principle, the equivalence between gravitational and inertial mass. The study was also one of the first to provide a measure of the expansion of the solar system by determining the mass loss of the Sun; the results that were found were very close to theoretical estimates of the Sun’s mass loss due to solar wind and processes in the Sun.
You have been chosen by NASA to study Phobos and Deimos, through JAXA’s MMX mission. What will your role be? What will you study and what do you expect to find?
I will use radio, camera, and laser altimeter data from MMX to determine the gravity field and rotation state of the moons Phobos and Deimos, and to verify models of their shape. Of course, MMX has its own science team who will be conducting a similar analysis. My project will use different tools and strategies, and will, in part, serve as a validation and verification of results. Knowing the mass, shape, and rotation state of Phobos is very important for the landing phase of the mission. My Co-Investigator, Andrew Liounis who is also from NASA Goddard Space Flight Center, and I will use the experience that we gained during the Origins, Spectral Interpretation, Resource Identification, Security-Regolith Explorer (OSIRIS-REx) mission to the asteroid 101955 Bennu. We did a similar analysis there and were able to provide greatly improved estimates of shape and mass. We focused especially on using differential image data, which can be thought of as images of the same reference point on the surface taken at different times. Our analysis for OSIRIS-REx showed that this can greatly improve the estimates of important parameters. We will do a similar analysis for MMX.
One of the main goals of the MMX mission is to elucidate the origin of Mars’ moons. There are two leading theories: they are thought to be captured asteroids, or the result of a giant impact. MMX will collect data, and a sample from Phobos, to figure out their origin. Knowledge of the gravity field will help to establish the bulk density and composition; spatial variations in the gravity field may provide clues about its structure and to see if there are density inhomogeneities.
To be honest, I am not sure what to expect! A recent release of an image of Deimos along with some spectral data interpretations from the United Arab Emirates’ Hope mission indicates that Deimos may be similar to Mars, suggesting it may not be a captured asteroid. MMX’ data of both Phobos and Deimos will thus be very important to make the distinction. And as always, I expect we will find answers to some of the outstanding issues, as well as new questions.
Can the gravitational field of the moons or planets change over time, or does it always remain the same?
The gravity field of a body can certainly change over time. There are several different reasons why this would be the case. If there is another, relatively large, body nearby, a planet or moon will experience tidal forces. These cause the body to deform (even though they consist mainly of rock!) as it orbits the disturbing body. This introduces changes in the body’s gravity field that vary with time. By determining such time-varying changes in the gravity field from spacecraft tracking data we can learn something about the interior of a body; how big those changes in the gravity field are depends on how “deformable” the body is, and that tells us something about the interior structure. It also generates heat, and this tidal heating could very well supply the energy that would be needed to maintain sub-surface oceans in the outer solar system, at places like Jupiter’s moon Europa and Saturn’s moon Enceladus where we think oceans exists (not to mention other moons where this is possible).
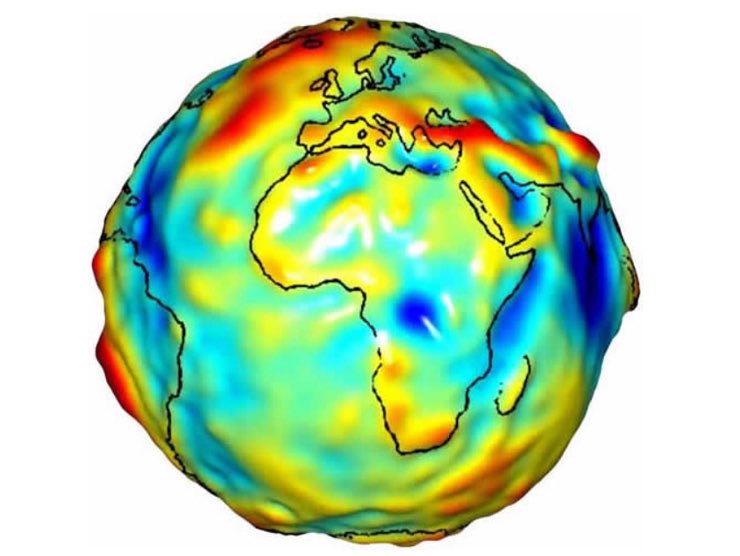
Credits: The University of Texas Center for Space Research/NASA Jet Propulsion Laboratory/GeoForschungsZentrum
Another reason why the gravity field would change is if there is some sort of mass exchange occurring on the planet. For the Earth, think of the oceans, and the water cycle. And especially, think of the shrinking ice masses at the poles and in Greenland. These changes are measured very precisely with the Gravity Recovery and Climate Experiment (GRACE) mission. In fact, the aforementioned GRAIL mission was heavily based on GRACE, the latter being the first to use the precise inter-satellite radio link to measure changes in the distance between two spacecraft in orbit around the Earth, from which changes in gravity could be determined precisely. Data from GRACE have shown the mass loss very precisely in Greenland, parts of Antarctica, and in other places.
But the Earth is not the only place where this is happening. Mars has an atmosphere, and there is a CO2 cycle that has the polar caps shrinking and growing. This can also be mapped using spacecraft tracking data, again by measuring time variations in the gravity field, and we routinely take this into account when analyzing Mars spacecraft data. And while Venus doesn’t have such a cycle, it has a thick atmosphere that causes changes in pressure at the surface, which in turn can change the gravity field.
Finally, the existence of an inner core that is slightly misaligned with respect to the rest of the planet may cause torques and as a consequence change the gravity field, again with a periodic signal. Depending on the size of the inner core, such changes can be small, but with good enough data, they can potentially be detected.
What do you love about your job? What has the Solar System taught you in life?
There are many things I love about my job. One of the main things is the opportunities that I have here at NASA to get involved in many different missions to many different solar system objects. I started out studying the Moon, and now have had the opportunity to work with data from spacecraft visiting other planets and moons: Mercury, Venus, Mars, Saturn’s moons Titan and Enceladus, Jupiter and its moons from the Juno project, and the asteroid Bennu. In addition, I get to work with the world’s experts on many different topics. I am not sure how to express what the Solar System itself has taught me in life. It’s such a wondrous place full of surprises. One really has to keep an open mind. There is always something new to learn, something to thwart your expectations and force you to rethink what you thought you knew.
- Cover image: Sander Goossens (central photo: science.gsfc.nasa.gov); side photo: JAXA YouTube (first image) / The University of Texas Center for Space Research/NASA Jet Propulsion Laboratory/GeoForschungsZentrum (second image)